Taming Chaos: IBM Quantum And Algorithmiq-led Scientists Report Today’s Quantum Computers Can Simulate Many-Body Quantum Chaos
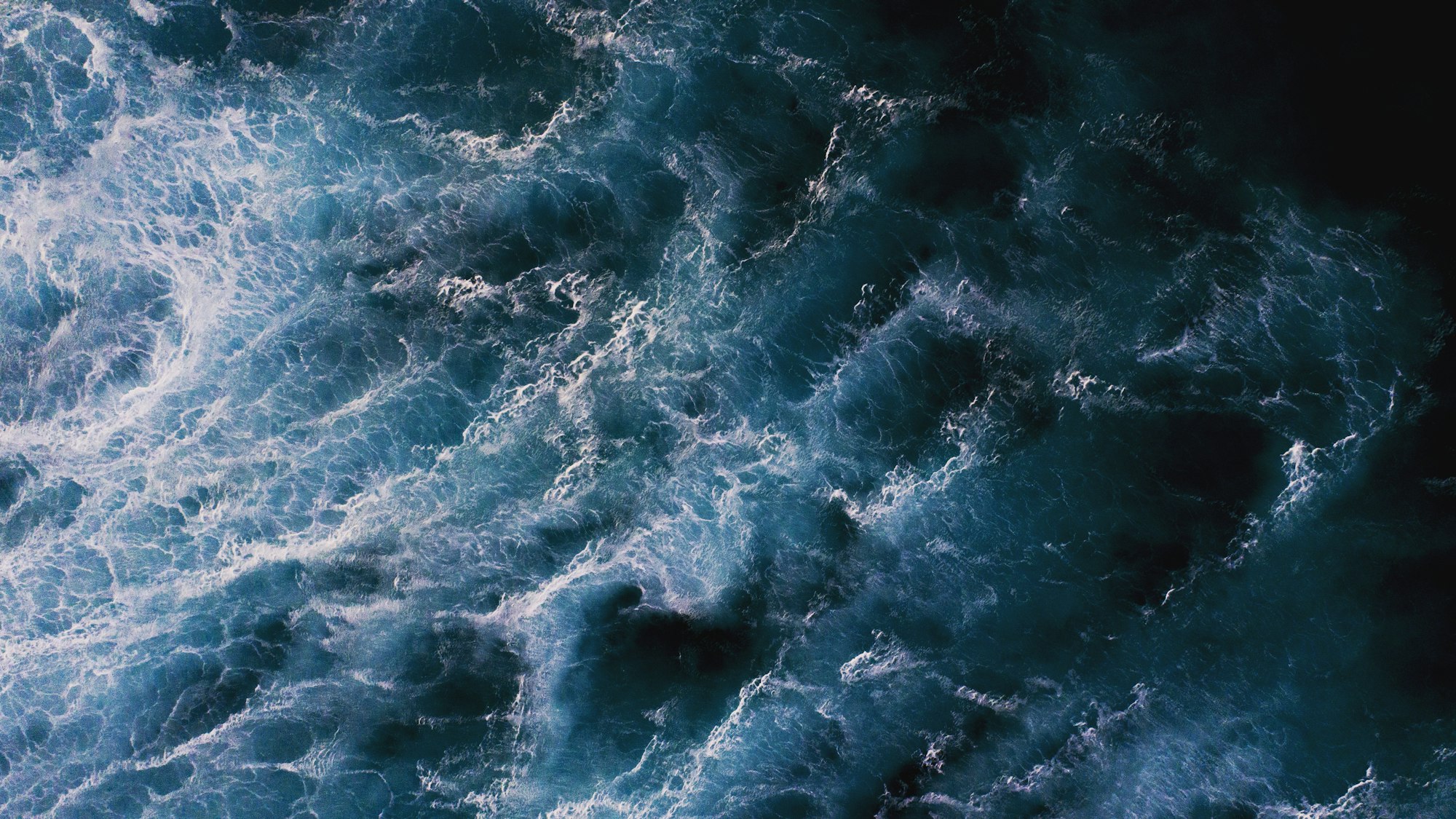
Insider Brief
- A recent study led by IBM Quantum used a quantum computer with up to 91 qubits to simulate many-body quantum chaos, an interaction-heavy process difficult for traditional computers to model.
- The research leveraged dual-unitary circuits to simulate chaotic behavior and employed tensor-network error mitigation to manage noise, enhancing the reliability of results.
- The findings suggest current quantum technology, though still developing, can address complex problems, with potential applications in weather prediction, fluid dynamics and material science.
Today’s quantum computers might be able to simulate complicated — often chaotic — behaviors found in nature, which could possibly lead to a better understanding of phenomena, such as complex weather patterns that are difficult to predict and biological systems that are important to health.
In a recent study, published in the pre-print server ArXiv, an IBM Quantum and Algorithmiq-led team of scientists used a quantum computer with up to 91 qubits to simulate a phenomenon called many-body quantum chaos, which is, essentially, the unpredictable behaviors observed in systems with many interacting particle. The sheer complexity of those interactions make it difficult to model using traditional computers. This effort, while still in the early stages of quantum computing development, point to how current quantum machines can be pushed to tackle problems that strain conventional technology, the scientists report.
Methods: Superconducting Transmon Qubits
The researchers used IBM’s “ibm_strasbourg” quantum processor, which operates with superconducting transmon qubits, known for their coherence properties that make them suitable for quantum experiments. The experiment was conducted using a type of quantum circuit known as a dual-unitary circuit. At the risk of being too simplistic, this is a specific way of designing quantum programs that can simulate chaotic behavior — an unpredictable evolution seen in systems with many interacting parts. These circuits were run on a quantum processor that used qubits, the basic units of quantum information that can represent more complex states than the typical bits used in classical computers.
Tensor-Network Error Mitigation
However, one of the major challenges with current quantum computers is noise, or errors, that can interfere with calculations. To counteract this, the researchers employed a technique called tensor-network error mitigation. This method involves using mathematical models to filter out the noise and correct the results of the quantum calculations, making them more reliable. The approach is crucial for getting meaningful data from today’s quantum machines, which are still far from being completely accurate.
The study didn’t stop at running the quantum simulations. The results were cross-checked against classical simulations that used powerful algorithms and methods to recreate the same chaotic behavior on traditional computers. These comparisons showed that while the quantum computer was consistent with the classical results for smaller system sizes, challenges emerged as the simulations grew more complex. This highlighted both the promise and current limitations of quantum computing.
Understanding quantum chaos is more than just an academic exercise; it has implications for how we use quantum computers in practical applications. Quantum chaos describes systems where small changes can lead to vastly different outcomes, a trait similar to what’s seen in chaotic weather systems or turbulent water. By exploring these types of behaviors with quantum computers, researchers can develop tools that may eventually help improve quantum error correction techniques or even enhance quantum-based cryptography and secure communications.
The scale of this study — working with up to 91 qubits and over 4,000 quantum operations — also points to the growing ability of quantum hardware to handle complex tasks.
The study’s findings could pave the way for more advanced simulations in areas like condensed matter physics, which studies how particles behave when they interact in dense materials. These insights could inform the design of new materials or help scientists better understand natural phenomena that are difficult to predict.
Limitations
Researchers noted some limitations in the work. First, although one of the key takeaways from the study is that quantum computers can now simulate complex processes, however they are not yet capable of doing so perfectly. The noise in these systems remains a hurdle.
The noise modeling, while managed with error mitigation, still presented challenges, especially at larger scales. The quantum circuits used were complex, but not immune to the imperfections of current technology. This highlights the importance of continued development in both quantum hardware and techniques for managing errors.
Even so, the use of error mitigation showed that researchers could push current hardware to achieve useful outcomes. This progress is important as it suggests that even before fully fault-tolerant quantum computers are built, today’s machines can still offer valuable insights into complicated problems.
Future research will likely focus on refining noise management and pushing for even greater accuracy in quantum simulations. As quantum hardware continues to improve, these experiments could be expanded to model even more complex systems. This would provide deeper insights not only for quantum computing itself but also for fields like cryptography, where understanding chaotic behavior can help create more secure systems.
The research team also suggested that quantum computers could one day be used to help design better quantum computers. This “feedback loop” of using quantum simulations to model and predict the behavior of quantum components could accelerate the development of more reliable and capable machines.
It’s important to note that the researchers published their findings in a pre-print server, which means the work has not been officially peer reviewed. For a deeper and more technical look at the work, which this story cannot provide, please review the study on AriXiv.
The work was conducted by scientists from leading institutions including IBM Quantum, IBM Research Europe, Ecole Polytechnique Fédérale de Lausanne, Algorithmiq Ltd, IBM Research, Trinity College Dublin, Università degli Studi di Milano, HUN-REN Wigner Research Centre for Physics, and Trinity Quantum Alliance.