Superconducting Qubit Baths Give Clean Simulation of Quantum Transport
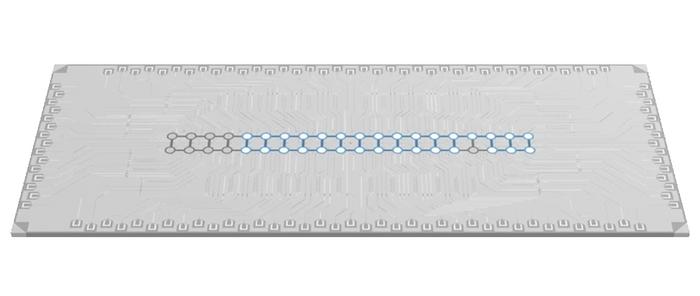
Insider Brief
- Researchers used a 31-qubit superconducting quantum processor to investigate quantum transport, revealing new insights into particle flow and thermalisation dynamics between quantum systems.
- The study, published in Nature Communications, demonstrated how differences in magnetisation between qubit groups drive steady spin currents, showcasing the versatility and precision of modern quantum simulators in the NISQ era.
- By leveraging theoretical models and experimental techniques, the team observed how larger quantum systems exhibit macroscopic behavior, offering a unified framework to understand nonequilibrium quantum phenomena.
- Image: Fabricated at the Micro-Nano Fabrication Centre of Zhejiang University, the 31-qubit superconducting quantum processor used in the experiment allows the researchers to engineer different types of transport and control the individual qubits. (Nature Communications 15, 10115, 2024)
PRESS RELEASE — Researchers from Singapore and China have used a superconducting quantum processor to study the phenomenon of quantum transport in unprecedented detail.
A better understanding of quantum transport, which can refer to the flow of particles, magnetisation, energy or information through a quantum channel, could propel advances in technologies such as nanoelectronics and thermal management.
“We’re quite excited because this is, practically, a new paradigm of doing quantum transport experiments,” says Centre for Quantum Technologies (CQT) Fellow Dario Poletti, whose co-corresponding authors for the new work published in Nature Communications on 22 November 2024 are Professor Haohua Wang from Zhejiang University (ZJU) and Professor Jie Hao from the Institute of Automation at the Chinese Academy of Sciences (CAS). He added, “We can now access information that we could not before with other previous implementations of quantum transport.”
Dario, who is also an Associate Professor and Head of Cluster at Singapore University of Technology and Design (SUTD), and a researcher under the MajuLab International Research Laboratory, derived theoretical models of quantum transport with Dr Xiansong Xu and Dr Chu Guo, back when they were both PhD students in SUTD. Xiansong is now an Assistant Professor at Sichuan Normal University while Chu Guo is an Assistant Professor at Henan Key Laboratory of Quantum Information and Cryptography. They tested these models with experimentalists from ZJU and CAS.
Experiments performed on the ZJU team’s 31-qubit quantum processor explored how a spin/particle current flows between two groups of qubits.
“The work also shows the usefulness of quantum simulation in the NISQ era,” says Pengfei Zhang, a Postdoctoral Fellow at ZJU. Pengfei is the co-first author of the publication together with Yu Gao, a PhD student at ZJU, and Xiansong. NISQ refers to noisy intermediate-scale quantum devices.
A unified picture
Quantum transport happens when there is some imbalance or nonequilibrium between systems in contact. For example, a temperature difference results in heat flow until systems are thermalised, while voltage differences result in a current.
The researchers studied transport between two groups of qubits with different magnetisation. In one group, or bath, all the qubits were initialised to be spin-down, while in the other bath, half the qubits were spin-down and the other half spin-up, averaging zero magnetisation. The two baths were connected by a point contact: a weak link between two qubits, one from each bath.
The theorists’ idea was to use concepts from quantum thermalisation to explain this quantum transport. They considered the two baths as a composite system that over time would thermalise. During this long process, they predicted, steady transport would occur.
Xiansong says, “I believe there should exist a unified picture of thermalisation dynamics and nonequilibrium steady dynamics. But both the theoretical derivation and numerical verifications are not straightforward.”
Observing transport
For the experimental group, the theoretical proposal seemed straightforward to implement. Because they have good individual control over every qubit in their quantum processors, they could set up different baths and so engineer different types of transport. Previous experiments on quantum transport did not have as much versatility or tunability.
The researchers studied how differences in the initial bath states and the number of qubits affected the scale and steadiness of the current.
The initial bath states could differ in exactly which qubits in the half-half configuration were spin-up and which spin-down. The researchers prepared 60 randomly chosen distinct initial states for systems of 14, 17 and 31 qubits, then measured the current after 200 nanoseconds. The distribution showed that the current converges towards the same value as the system size grows.
“This is sometimes called ‘typicality’,” says Dario. “All that matters is the average spin polarisation, a macroscopic quantity, not the details of the individual qubits or how they are prepared.”
The researchers also evaluated the steadiness of the current by measuring temporal fluctuations, appearing as spin flow to and fro between the baths. This involved 60,000 measurements at five-nanosecond intervals from 100 to 1,000 nanoseconds. They observed that the fluctuations became significantly smaller compared to the main signal as the system size grew, manifesting the emergence of the expected macroscopic physics.
Pengfei says, “It became challenging to fine-tune the control parameters and precisely measure the tiny temporal fluctuation of particle current for a large system, but we overcame it by developing a calibration protocol and an error mitigation method.”
The researchers hope to build on these results, and continue their collaboration to explore richer transport scenarios.