Scientists Report Superconducting Qubit Hits 72 GHz, Offering Path to Scalable Quantum Systems
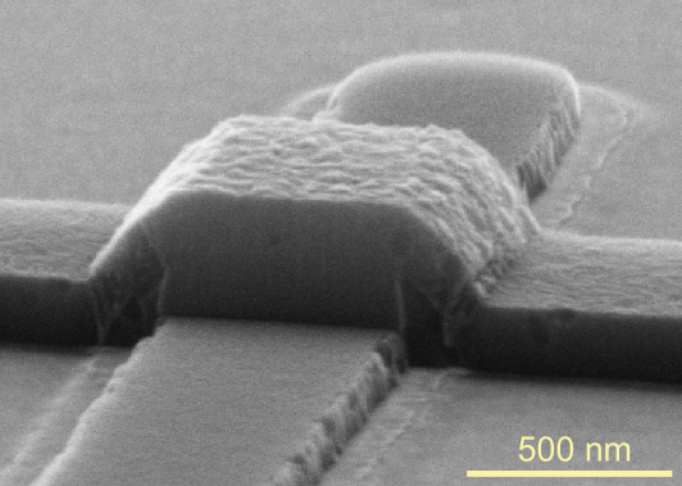
Insider Brief
- Researchers demonstrated qubits with coherence times of approximately 1 microsecond at frequencies up to 72 GHz, operating reliably at temperatures up to 250 millikelvin—substantially higher than the sub-50 millikelvin temperatures required by aluminum-based qubits.
- Higher operating temperatures reduce the cooling infrastructure’s complexity and cost, potentially paving the way for larger quantum systems.
- Future work could focus on improving fabrication processes, exploring even higher frequencies, and expanding applications to hybrid systems and quantum sensing.
Researchers have demonstrated a superconducting qubit operating at 72 GHz, work that could lead to enhanced scalability and a reduction in the costs of quantum computing systems.
The study, conducted by scientists at the University of Chicago and Stanford University and recently published on the pre-print server ArXiv, also showed that these qubits can function at temperatures up to 250 millikelvin, significantly higher than the sub-50 millikelvin temperatures required for most superconducting qubits.
According to the researchers, the advance is a step forward in addressing two major challenges in quantum computing: scaling the number of qubits in a system and managing the heat produced during their operation. By enabling qubits to operate at higher temperatures, the study suggests that quantum processors could require less extreme cooling, reducing both hardware overhead and energy costs.
The team writes: “By investigating transmon qubits at higher temperatures and frequencies than before, we gain a new perspective on superconducting qubit coherence. Our devices highlight the importance of increased frequency, which could even help improve aluminum microwave device performance up to ∼180mK, relaxing the strict thermalization requirements of current qubits, as well as exploring a new regime for studying physics with qubits in a conventional material platform.”
Niobium Trilayer is Key
The researchers developed transmon qubits using niobium trilayer Josephson junctions instead of the aluminum junctions commonly used in superconducting qubits. The niobium-based design demonstrated resilience to thermal noise and quasiparticle-induced decoherence, maintaining coherent operation up to 1 kelvin. At 250 millikelvin, the qubits exhibited performance metrics comparable to aluminum-based designs at much colder temperatures.
“The key element at the heart of our transmon qubit is a niobium trilayer Josephson junction with high critical current density,” the team writes.
The high frequency of the qubits, reaching up to 72 GHz, reduces their sensitivity to thermal noise and expands the range of possible interactions with other quantum systems. These properties make them particularly suited for hybrid quantum applications, where systems with varying thermal and electromagnetic characteristics must coexist.
Scalable and Efficient Quantum Computing?
Critics often charge that superconducting quantum computing approaches are held back by scalability issues. However, in this work, the work shows a path toward the ability to operate at higher temperatures, which could alleviate a key limitation in scaling these quantum computers. Current systems require dilution refrigerators to keep qubits at near absolute zero, a method that becomes less effective and more costly as the number of qubits increases. The increased cooling power available at higher temperatures means more qubits could be integrated into a single system without overwhelming the cooling infrastructure.
Additionally, higher-frequency qubits open the door to experiments involving new energy scales, enhancing the potential for quantum sensing and hybrid quantum systems. These systems, which combine different types of quantum emitters or couple quantum and classical components, often require qubits with both thermal resilience and high frequencies.
Leveraging Niobium and Optimized Fabrication
The study’s results were achieved, as mentioned, using niobium trilayer junctions, which have a higher superconducting transition temperature than aluminum. This property reduces the density of quasiparticles. This reduction helps because the quasiparticles are actually disturbances in a superconducting material that behave like particles, but can disrupt the coherent behavior of qubits. They arise when superconductivity breaks down locally, often due to thermal energy, and are a major source of decoherence in quantum systems.
To fabricate the qubits, the researchers used precision optical lithography and chemical treatments to eliminate surface defects that could degrade performance. These niobium-based qubits were then tested for coherence properties across a wide range of frequencies and temperatures. The devices maintained coherence times of about 1 microsecond, which is comparable to existing aluminum-based designs despite operating at significantly higher temperatures.
Higher operating frequencies further contributed to thermal resilience, as the qubits were less affected by environmental noise. This approach minimizes the need for active cooling measures, such as qubit reset mechanisms, simplifying system design.
Limitations and Challenges
The researchers indicated there are some limitations with the study and areas for future work. The qubits’ coherence times, while sufficient for many applications, are shorter than the best results achieved with aluminum-based designs at lower temperatures. Future work will need to refine fabrication techniques to enhance coherence further.
The integration of high-frequency qubits into existing quantum computing architectures might also present challenges. Hardware capable of supporting the higher frequencies required by these qubits is less mature and may increase the complexity of initial implementations.
Future Work: Higher Frequencies and Broader Applications?
The findings suggest pathways for even higher-frequency qubits. Niobium-based junctions have the potential to operate at frequencies as high as 700 GHz, offering access to new regimes in quantum experimentation. Future research will explore optimizing the qubit fabrication process to reduce losses and improve overall performance.
The study also suggests that thermal resilience could benefit other quantum technologies, including aluminum-based qubits, by adopting higher-frequency designs. Researchers plan to investigate how such modifications might relax the strict cooling requirements currently imposed on quantum systems.
In addition to improving scalability, the results open opportunities for hybrid quantum systems and experiments that demand greater heat dissipation. These include applications in quantum sensing, optical transduction, and simulations of high-energy physics.
The research team comprised Alexander Anferov from the University of Chicago’s James Franck Institute and Department of Physics, along with David I. Schuster, Shannon P. Harvey, and Fanghui Wan, all affiliated with Stanford University’s Department of Applied Physics and SLAC National Accelerator Laboratory. Jonathan Simon, also from Stanford’s Department of Physics, collaborated on the work.
ArXiv is a pre-print server, which means the work has not been technically peer-reviewed. However, the study is rigorous and technical. Please review it for a deeper, more technical dive into the study.