Researchers ‘Drop’ New Chemical Detection System That Unites Quantum Sensing With Microfluidics
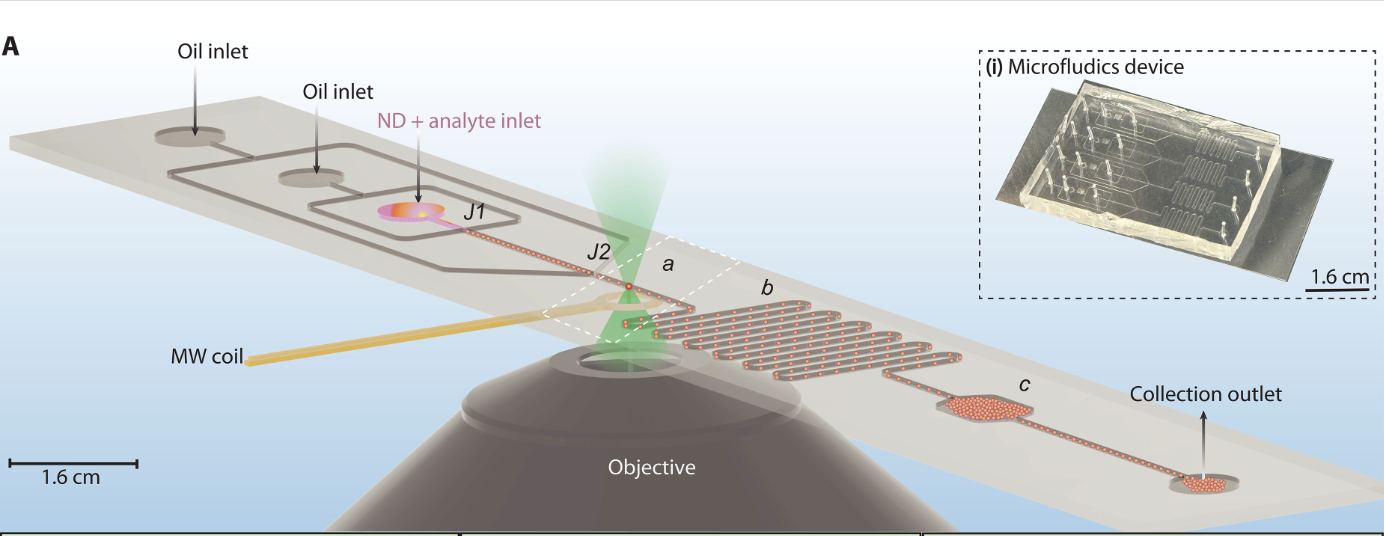
Insider Brief
- Researchers have developed a highly sensitive chemical detection system by combining quantum sensing with droplet microfluidics, enabling precise measurements with minimal sample volumes.
- The platform uses nanodiamonds containing nitrogen-vacancy (NV) centers encapsulated in microscopic liquid droplets, which improve measurement stability, reduce noise, and lower costs compared to single-crystal diamond sensors.
- Demonstrating detection limits as low as 100 nanomolar for paramagnetic ions, the system holds promise for applications like portable diagnostics, single-cell analysis, and environmental monitoring.
- Institutions involve include the University of California, Berkeley, Lawrence Berkeley National Laboratory, Adamas Nanotechnologies Inc., and the CIFAR Azrieli Global Scholars Program in Canada.
Researchers have combined quantum sensing and droplet microfluidics to develop a highly sensitive chemical detection system, offering applications from bioengineering to environmental monitoring.
The study, published in Science Advances, introduces a platform that uses quantum sensors made of nanodiamonds to detect chemicals with exceptional precision. These nanodiamonds, containing nitrogen-vacancy (NV) centers, are encapsulated in microscopic liquid droplets, allowing researchers to overcome key challenges in traditional chemical sensing. The novel approach reduces noise, enables stability over long durations, and requires minimal sample volumes.
“Important advances are enabled by this work, including portable chemical testing devices, amplification-free chemical assays, and chemical imaging tools for probing reactions within microenvironments,” the researchers note in the study.
What It Means
According to the team, the system’s main innovation lies in its ability to perform highly accurate chemical detection using incredibly small amounts of analyte, or test material. The technology could one day be used to develop portable chemical sensors, improve cellular analysis tools, and enable amplification-free chemical tests for a range of fields, including diagnostics, bioreactors, and environmental sensing.
The platform also holds promise for real-time intracellular monitoring, single-cell analysis and chemical imaging. By leveraging the precision of quantum sensors and the versatility of droplet microfluidics, the study opens new possibilities for probing reactions in confined environments.
For instance, the researchers propose that integrating this technology with flow cytometry — a technique for analyzing cells in fluid — could revolutionize single-cell metabolomics. By detecting reactive oxygen species (ROS) inside individual cells, the system may help monitor cellular metabolism with unprecedented detail.
Quantum Sensors in Droplets
Quantum sensing relies on nitrogen-vacancy (NV) centers in diamonds. These centers are defects where two carbon atoms are replaced by a nitrogen atom and an empty space, or vacancy. NV centers are particularly useful because their spin state — which relates to their magnetic properties — is highly sensitive to environmental changes. This sensitivity is captured by measuring shifts in the light emitted by the NV centers when exposed to microwaves and lasers.
Until now, chemical sensing with NV centers has primarily relied on single-crystal diamonds, which are expensive, require precise alignment, and offer limited surface interaction with target molecules, according to the researchers. In contrast, in this study, the team employs nanodiamonds — tiny diamond particles a few nanometers in size — that can interact closely with chemicals in a liquid environment. Nanodiamonds do not interact chemically or biologically with living tissue — bio-inert. They are also cost-effective and can be tailored for specific applications.
To improve performance, the researchers used droplet microfluidics, a method that manipulates tiny droplets of liquid in channels smaller than the width of a human hair. These droplets act as microscopic reaction chambers, confining the nanodiamonds and analytes in controlled conditions. By flowing the droplets through a detection system, researchers achieved stable, noise-resistant measurements over thousands of droplets.
How It Works
The system uses nanodiamonds loaded into picoliter-sized droplets — about a billion times smaller than a standard water droplet. The droplets flow through a microfluidic device where they are illuminated with green laser light and subjected to microwave fields.
The NV centers in the nanodiamonds emit red fluorescence, which changes depending on the presence of chemicals such as paramagnetic ions. This optical signal is detected and analyzed with a technique called optically detected magnetic resonance (ODMR).
Droplet movement averages out fluctuations caused by differences in nanodiamond size, orientation, or other inconsistencies, improving measurement accuracy. The researchers also employed a dual modulation technique — using microwaves and droplet flow — to isolate the quantum signal from background noise, further enhancing sensitivity.
In their experiments, the team demonstrated the system’s ability to detect gadolinium ions, a type of paramagnetic analyte, with a detection limit as low as 100 nanomolar, which the team writes is a level previously difficult to achieve in such small sample volumes. They also measured reactive species like TEMPOL, a common chemical probe for ROS, reaching detection limits below two micromolar.
Implications and Applications
The scientists write that their innovation paves the way for next-generation chemical sensing devices that are portable, precise, and low-cost. Potential applications include:
- Diagnostics: Amplification-free tests for detecting trace chemicals or biomarkers in blood and other fluids.
- Bioengineering: Real-time monitoring of cellular metabolism or chemical reactions in bioreactors.
- Environmental Monitoring: Field-deployable devices for detecting pollutants or contaminants in water and air.
- Single-Cell Analysis: Tools for studying individual cells’ chemical makeup, offering insights into metabolism, disease, and drug response.
The integration of this platform with existing technologies, such as flow cytometry, could significantly expand how it can be used. Researchers envision a “quantum-enhanced” version of flow cytometry that combines cellular analysis with precise chemical sensing.
Study Limitations and Future Directions
The platform’s current capabilities are limited to specific analytes like gadolinium and TEMPOL. Future studies will need to explore broader chemical detection ranges and validate the system for real-world applications.
The researchers also aim to develop methods for tracking nanodiamonds within droplets, enabling real-time chemical imaging. They also propose improving throughput — analyzing millions of droplets per hour — and integrating the system into compact, portable devices for field use.
Another potential avenue is the use of nanodiamonds for advanced applications like bulk magnetometry or accelerometers. The researchers highlight that the platform’s stability over hours of continuous measurement makes it ideal for such high-precision tasks.
The study demonstrates how quantum technologies can merge with existing tools to deliver practical solutions. By addressing limitations in traditional chemical sensing, this approach offers a foundation for high-precision, cost-effective, and scalable detection systems.
Please read the research paper in Science Advances for a deeper, more technical look at the work.
The research was led by Ashok Ajoy at the University of California, Berkeley, and Lawrence Berkeley National Laboratory, with contributions from Adrisha Sarkar, Zachary R. Jones, Madhur Parashar, Emanuel Druga, Amala Akkiraju, Sophie Conti, Pranav Krishnamoorthi, Srisai Nachuri, Parker Aman, and Mohammad Hashemi, all affiliated with UC Berkeley. Zachary Jones and Deepti Tanjore are also associated with the Lawrence Berkeley National Laboratory’s Advanced Biofuels and Bioproducts Process Development Unit. Nicholas Nunn, Marco D. Torelli, and Olga A. Shenderova of Adamas Nanotechnologies Inc. provided resources and support. Benjamin Gilbert and Kevin R. Wilson, both from Lawrence Berkeley National Laboratory, contributed to study, as well.