PsiQuantum, Boehringer Ingelheim Researchers Report Speedup For Complex Molecular Simulations
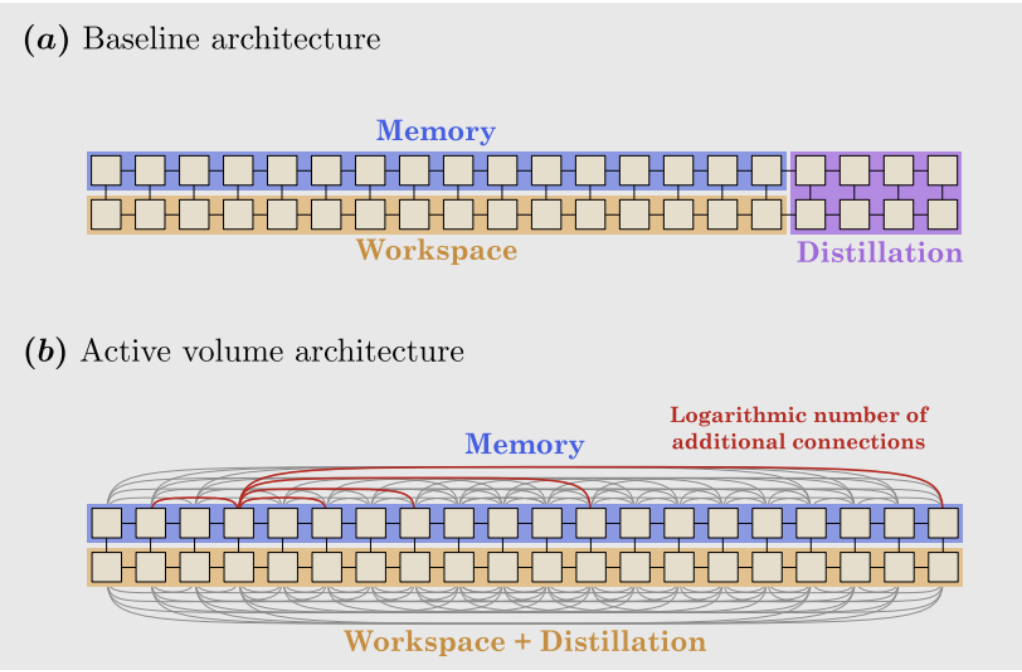
Insider Brief
- PsiQuantum and Boehringer Ingelheim researchers demonstrated a 234x and 278x speedup in calculating the electronic structures of Cytochrome P450 and FeMoco, respectively, using advanced quantum algorithms and specialized photonic hardware.
- The study employed a combination of BLISS and Tensor Hypercontraction methods, along with Active Volume architecture, to optimize calculations on fault-tolerant quantum computers.
- These advancements pave the way for practical quantum applications in drug design and materials science, though they currently rely on PsiQuantum’s specific photonic platform and FTQC technology still under development.
A new study led by PsiQuantum and Boehringer Ingelheim demonstrates a computational speedup to calculate the electronic structures of two challenging molecules, Cytochrome P450 and FeMoco, using quantum computing methods. The research, published on the pre-print server arXiv, introduces techniques that make these calculations 234 to 278 times faster, signaling progress toward industrial relevant quantum applications.
The molecules targeted in this study are vital to fields such as drug metabolism and agricultural chemistry. Cytochrome P450, for example, plays a key role in breaking down drugs in the human body, while FeMoco, part of the nitrogenase enzyme, is essential for nitrogen fixation, a critical process for agricultural fertilizers. Calculating these molecules’ electronic structures has long been computationally expensive due to their complexity.
The study found that PsiQuantum’s photonic quantum hardware and new algorithmic techniques, including an Active Volume (AV) hardware architecture and methods like BLISS (Block-Invariant Symmetry Shift) and Tensor Hypercontraction (THC), drastically reduce runtime. For Cytochrome P450, the speedup factor was 234 times; for FeMoco, 278 times.
For clarity, speedup factor refers to the reduction in computational time achieved — in the case of this study — by combining advanced quantum algorithms, optimized circuit designs and a specialized hardware architecture compared to previous methods for calculating the electronic structure of molecules.
Methodology
According to the paper, PsiQuantum’s approach involves combining advanced hardware design with innovative algorithms:
- Active Volume (AV) Architecture: This method leverages photonic qubits in a highly interconnected layout, eliminating bottlenecks common in quantum systems and enabling parallel operations.
- BLISS and THC Techniques: These techniques reduce the mathematical complexity of calculations by compressing data and exploiting symmetries in the molecular systems. BLISS incorporates symmetry shifts to minimize redundant calculations, while THC simplifies multi-body interactions.
- Circuit Optimization: Refining quantum circuits further reduced computational overhead, contributing a 10% additional speedup.
It was the clever integration of these innovations that created a scalable system capable of tackling highly correlated electronic structures with unprecedented efficiency.
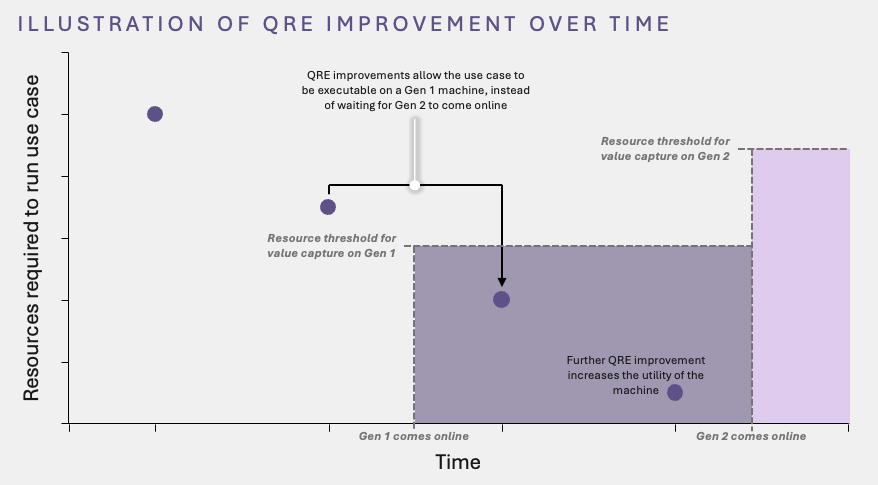
Electron Interaction And Exponential Scaling
Electronic structure calculations determine how electrons interact within a molecule, a task critical for drug design, catalyst development and materials science. Classical computers struggle with such tasks for complex systems due to exponential scaling, forcing reliance on approximations. Quantum computing promises exact solutions, however early quantum systems were too slow for practical use.
The innovations in this study signal a shift in the ability to use quantum computers for practical — and industrial relevant — uses. By employing AV-compatible quantum hardware and efficient algorithms, researchers achieved runtimes that align with industrial workflows. For example, simulations that once required days can now be completed in hours or less, the paper suggests.
Limitations And Future Work
This is early work and there are a number of factors researchers will need to address. Current quantum systems, even at their most advanced, may not be able to achieve these results at scale. The methods also require precise control over quantum operations, demanding high-quality error correction and stable qubits.
The study’s authors emphasize the need for further optimization and hardware advancements to make these techniques widely applicable. Immediate next steps include refining error correction protocols, scaling up hardware capacity, and exploring additional chemical systems. PsiQuantum and its partners plan to expand their focus beyond Cytochrome P450 and FeMoco to other molecules relevant to drug design and industrial catalysis. They also aim to develop methods that integrate seamlessly with hybrid quantum-classical workflows.
In a blog post on the work, the team adds: “While significant, this result is not the final step in speeding up calculations. There are further improvements that can be made in the preparation of the Hamiltonian before we run into theoretical limits, e.g., the 1-norm of the Hamiltonian is still ~2x higher than the limit for the Cytochrome P450 case. Moreover, the AV architecture can bear further scrutiny because the current result assumes upper bounds on subroutines in the algorithm, representing worst-case estimates, meaning that a tighter analysis of AV costs would have an immediate impact on the speedup without changes to the algorithm itself. Working on these and other possible improvements is the next target, and Boehringer Ingelheim and PsiQuantum look forward to the collaboration that will enable these improvements.”
The researchers also write in the study: “We are optimistic that the resource estimates in this work will be improved. To this end, we depend on the contributions of many disciplines on all levels of the quantum computing stack. The impact of novel developments in quantum error correction, for instance, would need to be considered within the cost model. By continuously improving the cost of this use-case, it is our aim to bring practical quantum computing closer and to contribute to the path to industrial value.”
In the future if the work is verified, the findings could have significant implications for industries like pharmaceuticals, agriculture and energy are significant. With the ability to accelerate calculations, pharmaceutical researchers could shorten the timeline for discovering new drugs — which could lower drug prices and open up new avenues to personalized medicine — or it could help scientists design efficient catalysts. In agriculture, improved understanding of nitrogen fixation could lead to more sustainable fertilizer production, which would impact the environment.
Often, studies on quantum computing advances, can be superficially centered solely on the hardware. This study not only showcases the potential of quantum hardware but also reveals the importance of collaboration between algorithm designers, hardware developers and industry stakeholders.
The researchers involved in this study include Athena Caesura, Cristian L. Cortes, William Pol, Sukin Sim and Mark Steudtner, all from PsiQuantum. From the Quantum Lab at Boehringer Ingelheim, the contributors are Gian-Luca R. Anselmetti, Matthias Degroote, Nikolaj Moll, Raffaele Santagati and Michael Streif.
The paper itself is highly technical — and this article attempts a basic overview — however, for further questions or a deeper dive into the techniques, you may want to read the paper, itself. Please note: papers on pre-print servers have not been formally peer-reviewed. Researchers use these online platforms to receive immediate — but informal — feedback on their work.