Innovative Quantum Processor Utilizes Fermionic Atoms for Efficient Simulation of Complex Physical Systems
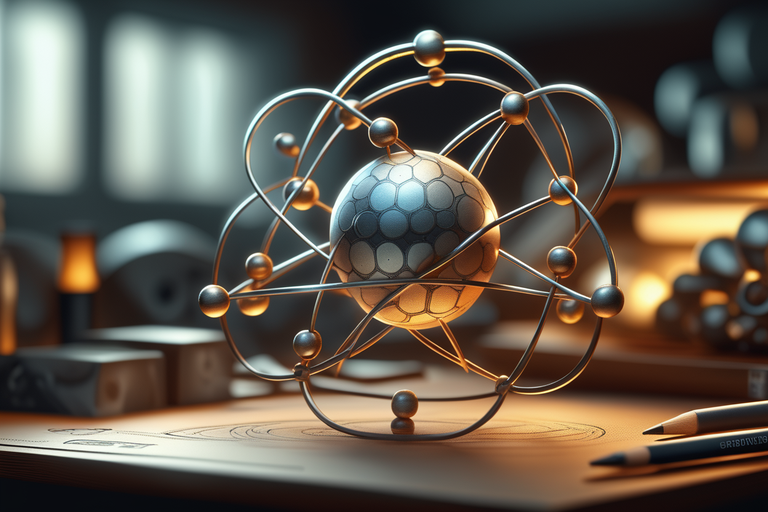
Insider Brief
- Austrian and US researchers have created a quantum computer using fermionic atoms to replicate intricate physical systems.
- This quantum processor employs programmable neutral atom arrays and fermionic gates to simulate fermionic models efficiently.
- Peter Zoller’s team from at the Institute for Quantum Optics and Quantum Information (IQOQI) of the Austrian Academy of Sciences showcased the processor’s effectiveness in accurately emulating fermionic models from quantum chemistry and particle physics.
Researchers hailing from Austria and the United States have introduced a novel form of quantum computer that employs fermionic atoms to replicate intricate physical phenomena. This processor harnesses programmable neutral atom arrays to facilitate the simulation of fermionic models in a hardware-efficient manner, utilizing specialized fermionic gates. Under the leadership of Peter Zoller, the team has successfully demonstrated the prowess of this quantum processor, showcasing its effectiveness in simulating fermionic models originating from domains like quantum chemistry and particle physics.
A closer look into fermionic atoms reveals their adherence to the Pauli exclusion principle, which stipulates that two of these atoms cannot simultaneously occupy the same quantum state. This characteristic renders them particularly suitable for emulating systems where fermionic statistics wield significant influence, including molecules, superconductors, and quark-gluon plasmas.
“In qubit-based quantum computers, supplementary resources must be allocated to simulate these attributes, often manifesting as extra qubits or elongated quantum circuits,” said Daniel Gonzalez Cuadra from the research group led by Peter Zoller at the Institute for Quantum Optics and Quantum Information (IQOQI) of the Austrian Academy of Sciences (ÖAW) and the Department of Theoretical Physics at the University of Innsbruck, Austria.
Diving into Fermionic Quantum Processing
The foundation of a fermionic quantum processor rests on a fermionic register alongside an assemblage of fermionic quantum gates. Gonzalez Cuadra delineated: “The register comprises an array of fermionic modes, which can be either unoccupied or occupied by a single fermion, with these two states constituting the elemental unit of local quantum information. The envisaged system’s state, such as a molecule composed of multiple electrons, generally takes the form of a superposition of numerous occupation patterns, all of which can be directly encoded within this register.” Subsequently, this information undergoes processing through a fermionic quantum circuit, meticulously crafted to imitate phenomena like the time evolution of a molecule. These circuits distill into sequences containing two primary fermionic gate types: tunnelling and interaction gates.
The researchers propose confining fermionic atoms within an array of optical tweezers—focused laser beams engineered to accurately manipulate atoms. “This platform intrinsically supports the complete set of required fermionic quantum gates: tunnelling gates transpire via atom tunnelling between two optical tweezers, while interaction gates materialize through initial atom excitation to high-dipole Rydberg states,” said Gonzalez Cuadra.
Spanning Quantum Chemistry to Particle Physics
Fermionic quantum processing assumes particular relevance in the simulation of systems featuring numerous interacting fermions, encompassing scenarios like electrons within molecules or materials, and quarks confined within protons. Its applicability spans diverse fields, spanning from quantum chemistry to particle physics. The research team effectively exhibits how their fermionic quantum processor adeptly emulates fermionic models arising within quantum chemistry and lattice gauge theory — two intricate realms of physics traditionally challenging to tackle using classical computers.
Gonzalez Cuadra reflects on the strengths of fermionic quantum processing, noting: “By utilizing fermions to encode and manipulate quantum information, certain attributes of the simulated system become inherently assured at the hardware level, a feat necessitating supplementary resources within conventional qubit-based quantum computers.” He envisions a bright future for the field, aiming to identify promising applications for fermionic quantum processing and devise tailored algorithms primed for deployment in near-term quantum devices.
The outcomes of the present research have been featured in the Proceedings of the National Academy of Sciences (PNAS). Financial support was provided by institutions like the Austrian Science Fund FWF, European Union, and Simons Foundation, among others. This development augments the landscape of quantum computing, paving the way for efficient simulations across a myriad of intricate physical systems.
Featured image: playground ai