Google’s Sycamore chip: no wormholes, no superfast classical simulation either
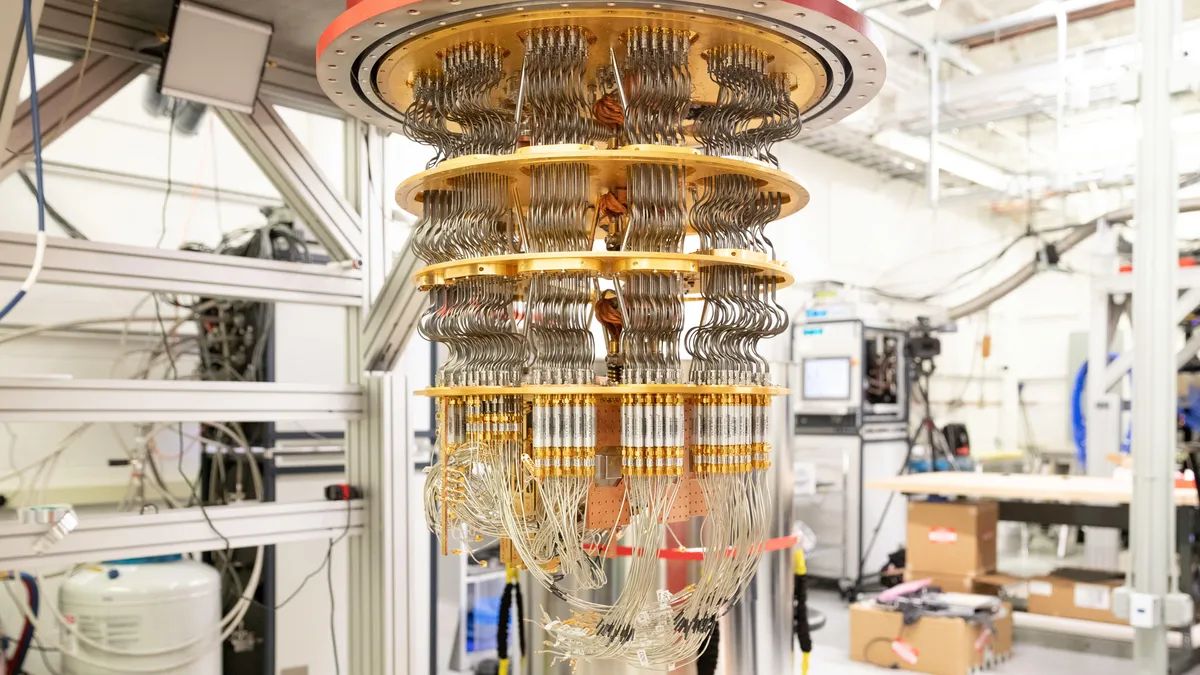
This is going to be one of the many Shtetl-Optimized posts that I didn’t feel like writing, but was ultimately given no choice but to write.
News, social media, and my inbox have been abuzz with two claims about Google’s Sycamore quantum processor, the one that now has 72 superconducting qubits.
The first claim is that Sycamore created a wormhole (!)—a historic feat possible only with a quantum computer. See for example the New York Times and Quanta and Nature (and of course, the actual paper), but also Peter Woit’s blog and Chad Orzel’s blog.
The second claim is that Sycamore’s pretensions to quantum supremacy have been refuted. The latter claim is based on this recent preprint by Dorit Aharonov, Xun Gao, Zeph Landau, Yunchao Liu, and Umesh Vazirani. No one—least of all me!—doubts that they’ve proven a powerful new technical result, thereby solving a significant open problem in the theory of noisy random circuit sampling. On the other hand, it might be less obvious how to interpret their result and put it in proper context. See also a YouTube video of Yunchao speaking about the new result at this week’s Simons Institute Quantum Colloquium, and of a panel discussion afterwards, where Yunchao, Umesh Vazirani, Adam Bouland, Sergio Boixo, and your humble blogger discuss what it means.
On their face, the two claims about Sycamore might seem to be in tension. After all, if Sycamore can’t do anything beyond what a classical computer can do, then how exactly did it bend the topology of spacetime?
I submit that neither claim is true. On the one hand, Sycamore did not “create a wormhole.” On the other hand, it remains pretty hard to simulate with a classical computer, as far as anyone knows. To summarize, then, our knowledge of what Sycamore can and can’t do remains much the same as last week or last month!
Let’s start with the wormhole thing. I can’t really improve over how I put it in Dennis Overbye’s NYT piece:
“The most important thing I’d want New York Times readers to understand is this,” Scott Aaronson, a quantum computing expert at the University of Texas in Austin, wrote in an email. “If this experiment has brought a wormhole into actual physical existence, then a strong case could be made that you, too, bring a wormhole into actual physical existence every time you sketch one with pen and paper.”
More broadly, Overbye’s NYT piece explains with admirable clarity what this experiment did and didn’t do—leaving only the question “wait … if that’s all that’s going on here, then why is it being written up in the NYT??” This is a rare case where, in my opinion, the NYT did a much better job than Quanta, which unequivocally accepted and amplified the “QC creates wormhole” framing.
Alright, but what’s the actual basis for the “QC creates wormhole” claim, for those who don’t want to leave this blog to read about it? Well, the authors used 9 of Sycamore’s 72 qubits to do a crude simulation of something called the SYK (Sachdev-Ye-Kitaev) model. SYK has become popular as a toy model for quantum gravity. In particular, it has a holographic dual description, which can indeed involve a spacetime with one or more wormholes. So, they ran a quantum circuit that crudely modelled the SYK dual of a scenario with information sent through a wormhole. They then confirmed that the circuit did what it was supposed to do—i.e., what any laptop could easily calculate that it would do ().
So, the objection is obvious: if someone simulates a black hole on their classical computer, they don’t say they thereby “created a black hole.” Or if they do, journalists don’t uncritically repeat the claim. Why should the standards be different just because we’re talking about a quantum computer rather than a classical one?
Did we at least learn anything new about SYK wormholes from thie simulation? Alas, not really, because 9 qubits take a mere 29=512 complex numbers to specify their wavefunction, and are therefore trivial to simulate on a laptop. There’s some argument in the paper that, if the simulation were scaled up to (say) 100 qubits, then maybe we would learn something new about SYK. Even then, however, we’d mostly learn about certain corrections that arise because the simulation was being done with “only” n=100 qubits, rather than in the n→∞ limit where SYK is rigorously understood. But while those corrections, arising when n is “neither too large nor too small,” would surely be interesting to specialists, they’d have no obvious bearing on the prospects for creating real physical wormholes in our universe.
And yet, this is not a sensationalistic misunderstanding invented by journalists. Some prominent quantum gravity theorists themselves—including some of my close friends and collaborators—persist in talking about the simulated SYK wormhole as “actually being” a wormhole. What are they thinking?
Daniel Harlow’s argument to me was that, if you had two entangled quantum computers, one on Earth and the other in the Andromeda galaxy, and if they were both simulating SYK, and if Alice on Earth and Bob in Andromeda both uploaded their own brains into their respective quantum simulations, then he believes they could have the subjective experience of jumping into a wormhole and meeting each other in the middle—something that wouldn’t have been possible with a merely classical simulation. Of course, this depends on some strong assumptions not merely about quantum gravity, but also about the metaphysics of consciousness—and even if it’s true, it’s still unclear to me that we shouldn’t call it a simulated wormhole for simulated people.
Alright, let’s move on to the claim that quantum supremacy has been refuted. What Aharonov et al. actually show, building on earlier work by Gao and Duan, is that Random Circuit Sampling, with a constant rate of noise per gate and no error-correction, can’t provide a scalable approach to quantum supremacy. Or more precisely: as the number of qubits n goes to infinity, and assuming you’re in the “anti-concentration regime” (which in practice probably means: the depth of your quantum circuit is at least ~log(n)), there’s a classical algorithm to sample the quantum circuit’s output distribution in poly(n) time (albeit, not yet a practical algorithm).
Here’s what’s crucial to understand: this is 100% consistent with what those of us working on quantum supremacy had assumed since at least 2016. We knew that if you tried to scale Random Circuit Sampling to 200 or 500 or 1000 qubits, while you also increased the circuit depth proportionately, the signal-to-noise ratio would become exponentially small, meaning that your quantum speedup would disappear. That’s why, from the very beginning, we targeted the “practical” regime of 50-100 qubits: a regime where you can still see explicitly that you’re exploiting a 250– or 2100-dimensional Hilbert space for computational advantage, thereby confirming one of the main predictions of quantum computing theory, but you also have a signal that (as it turned out) is large enough to see with heroic effort. To their credit, Aharonov et al. explain all this perfectly clearly in their abstract and introduction. I’m just worried that others aren’t reading their paper as carefully as they should be!
So then, what’s the new advance in the Aharonov et al. paper? Well, there had been some hope that circuit depth ~log(n) might be a sweet spot, where an exponential quantum speedup might both exist and survive constant noise, even in the asymptotic limit of n→∞ qubits. Nothing in Google’s or USTC’s actual Random Circuit Sampling experiments depended on that hope, but it would’ve been nice if it were true. What Aharonov et al. have now done is to kill that hope, using powerful techniques involving summing over Feynman paths in the Pauli basis.
Stepping back, what is the current status of quantum supremacy based on Random Circuit Sampling? I would say it’s still standing, but more precariously than I’d like—underscoring the need for new and better quantum supremacy experiments. In more detail, Pan, Chen, and Zhang have shown how to simulate Google’s 53-qubit Sycamore chip classically, using what I estimated to be 100-1000X the electricity cost of running the quantum computer itself (including the dilution refrigerator). Approaching from the problem from a different angle, Gao et al. have given a polynomial-time classical algorithm for spoofing Google’s Linear Cross-Entropy Benchmark (LXEB)—but their algorithm can currently achieve only about 10% of the excess in LXEB that Google’s experiment found.
So, though it’s been under sustained attack from multiple directions these past few years, I’d say that the flag of quantum supremacy yet waves. The Extended Church-Turing Thesis is still on thin ice. The wormhole is still open. Wait … no … that’s not what I meant to write…