Google Quantum AI Sees Quantum as Engine to Power Deep Tech Use Cases
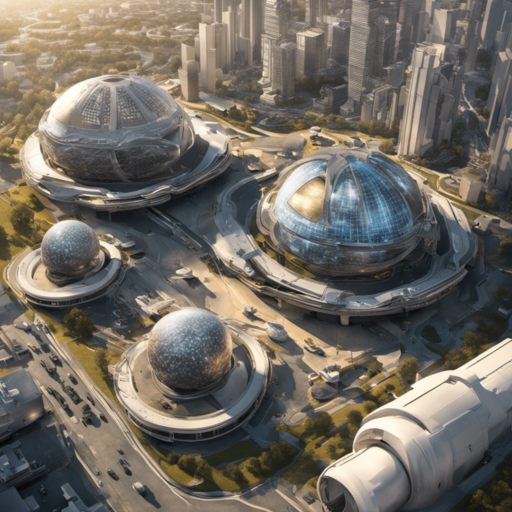
Insider Brief
- Quantum computing could help make tomorrow’s technologies more feasible.
- A Google Quantum AI team of scientists explore how quantum computers could be used to tackle improvements in biotechnology and new energy advances.
- The researchers say success won’t occur overnight, but progress on quantum algorithms is a step in that direction.
While most of the quantum industry is probing ways for the use of quantum computers to improve everyday tasks, Google Quantum AI released a blog post showing examples of how quantum may help power discoveries that would lead to future technologies and future breakthroughs. The researchers also offer estimates of just how much quantum power will be needed to bring these — once thought to be science fiction — technologies into reality.
In the post, written by Nicholas Rubin, senior research scientist, and Ryan Babbush, head of quantum algorithms, Quantum AI Team, the team sees that quantum computers can be important research partners for understanding drug metabolism, developing more effective lithium-ion batteries and designing real fusion reactors.
The researchers write that matching real-world — or even future-world — problems rests on finding the tasks quantum computers are good at tackling, while focusing on writing the correct quantum algorithms for that task.
They write: “We want to be more concrete: Exactly which problems are quantum computers more suited to tackle than their classical counterparts, and exactly what quantum algorithms could we run to solve these problems? Once we’ve designed an algorithm, we can go beyond analysis based on asymptotic scaling — we can determine the actual resources required to compile and run the algorithm on a quantum computer, and how that compares to a classical computation.”
The CYP450 Mechanism
According to Google Quantum AI, the pharmaceutical industry’s potential for harnessing the power of quantum computers has been a subject of great interest. However, concrete examples of how quantum computing could be applied have remained scarce. Collaborating with Boehringer Ingelheim, startup QSimulate, and academic experts from Columbia University, the blog writers explored one such example in their 2022 PNAS article titled “Reliably assessing the electronic structure of cytochrome P450 on today’s classical computers and tomorrow’s quantum computers.”
Cytochrome P450, a family of enzymes naturally occurring in humans, plays a pivotal role in drug metabolism, accounting for more than 70% of this crucial process. Yet, the intricate electron interactions within these enzymes have posed challenges for scientists, making it difficult to predict how effective they will be with different drugs.
In their paper, the blog writers outlined how quantum computing could address this complex issue. They began by determining the computational resources required to simulate CYP450’s intricate metabolic processes on classical computers. Then, they envisioned the implementation of a phase-estimation algorithm, essential for calculating the ground-state energies of electronic configurations throughout the reaction chain, on a surface-code error-corrected quantum computer.
Their findings showcased the potential of quantum computing to offer more accurate insights into the ever-changing electronic structures within CYP450 during drug metabolism while using fewer computational resources. Notably, the blog writers emphasized that the enhanced accuracy provided by quantum computers was not just beneficial but indispensable for unraveling the intricate chemistry involved. Furthermore, as the system’s complexity increased, quantum computers continued to outperform classical counterparts.
Ultimately, the blog writers concluded that achieving quantum advantage for this complex problem would need several million physical qubits. This study underscores the promising role of quantum computing in the pharmaceutical industry, shedding light on its potential to revolutionize drug development and metabolic research.
Lithium-ion Batteries
Lithium-ion batteries are crucial components of modern technology, but their reliance on cobalt in cathodes poses environmental and ethical concerns. Researchers have long sought alternatives, with lithium nickel oxide (LNO) emerging as a promising option. However, the intricacies of LNO’s chemical properties, especially its ground state, remained poorly understood. In their collaborative effort involving BASF, QSimulate, and Macquarie University, researchers published a paper titled “Fault tolerant quantum simulation of materials using Bloch orbitals.”
This work aimed to leverage quantum computing to simulate materials with periodic atomic structures like LNO. The researchers designed algorithms to study the relative energies of different LNO structures, a task deemed too expensive for classical computers. Their findings indicated that quantum computers would require tens of millions of physical qubits for accurate calculations, a goal that may become achievable with future algorithmic advancements.
Fusion Energy
In the realm of inertial confinement fusion (ICF) experiments, where extreme conditions prevail, simulations are computationally demanding and rely on uncertain material property models. Collaborating with Sandia National Laboratories and Macquarie University, researchers tackled the challenges of simulating the dynamics of charged particles in ICF experiments, such as those at the National Ignition Facility.
Their preprint, “Quantum computation of stopping power for inertial fusion target design,” introduced a quantum algorithm to compute stopping power in warm dense matter—a crucial factor in reactor efficiency. By understanding how high-energy alpha particles interact with surrounding plasma, researchers can optimize fusion reactor designs. While estimating the quantum algorithm’s resource requirements falls between previous applications, the researchers anticipate algorithmic improvements to reduce costs. This approach offers a more promising alternative to classical methods, which currently rely on mean-field approaches with inherent systematic errors in describing these complex systems.
The researchers suggest this is the first step — but an important first step in turning quantum power into real benefits. They conclude: “Developing and improving algorithms today prepares us to take full advantage of them when an error-corrected quantum computer is eventually realized. Just as in the classical computing case, we expect improvements at every level of the quantum computing stack to further lower the resource requirements. But this first step helps separate hyperbole from genuine applications amenable to quantum computational speedups.”