A Twisted Path to Innovation: Vortex Electric Fields in 2D Materials Advance Electronics and Quantum Devices
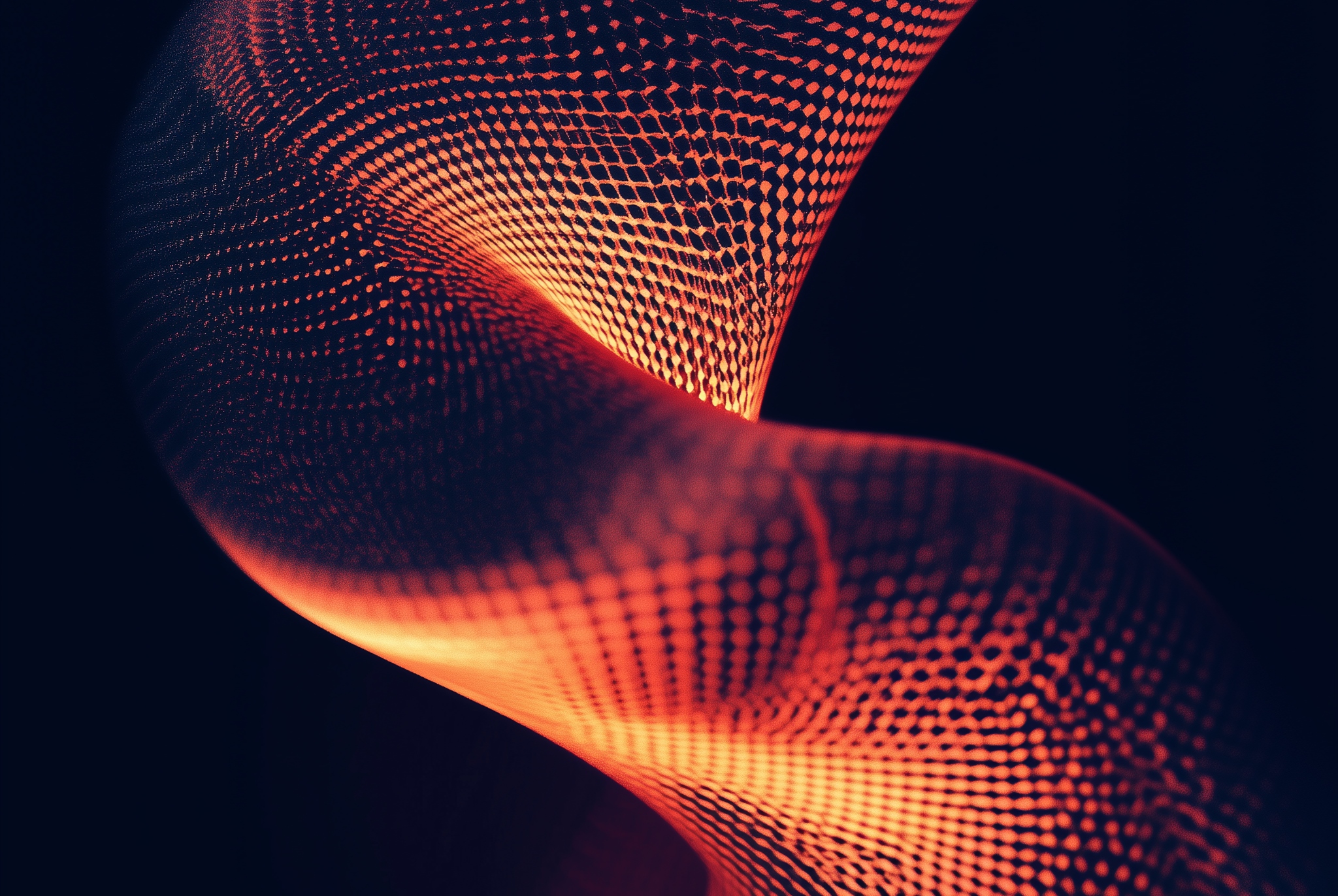
Insider Brief:
- Researchers at City University of Hong Kong discovered how twisting bilayer 2D materials creates a vortex electric field, relevant for advancements in quantum computing, spintronics, and nanotechnology by offering a cost-effective alternative to traditional fabrication techniques.
- The team developed an ice-assisted transfer technique, allowing precise control of twist angles and clean bilayer interfaces, necessary for optimizing electronic properties and enhancing quantum device performance.
- The discovery of quasicrystals in twisted bilayers introduces opportunities to improve memory stability, ultrafast computing speeds, and dissipationless spintronics.
In the world of science, even a small twist may carry immense implications for materials. Researchers at City University of Hong Kong have uncovered how a subtle rotation in 2D layers can give rise to a vortex electric field. This finding, published in Science, has the potential to impact electronic, magnetic, and optical devices as well as new applications in quantum computing, spintronics, and nanotechnology. According to Professor Ly Thuc Hue of CityUHK’s Department of Chemistry, the study demonstrates how “a simple twist in bilayer 2D materials” can induce this electric field, bypassing the need for costly thin-film deposition techniques.
Precision Techniques for New Potential in 2D Materials
Akin to solving intricate technical puzzles, researchers had to ensure clean, precisely aligned layers of material—a notoriously difficult challenge in the world of 2D materials. Twisted bilayers are made by stacking two thin layers of a material at a slight angle, creating unique electronic properties.
However, traditional methods of synthesizing these bilayers often limit the range of twist angles, particularly at smaller degrees, making exploration of their full potential nearly impossible. To address this, the team at City University of Hong Kong developed an ice-assisted transfer technique that uses a thin sheet of ice to align and transfer bilayers with precision.
This method not only allowed researchers to manipulate twist angles freely, ranging from 0 to 60 degrees, but also solved a long-standing issue of maintaining clean interfaces between layers. Clean interfaces are crucial because even minor impurities can disrupt the delicate electronic properties researchers are trying to observe.
To confirm the presence of the vortex electric field, the team used four-dimensional transmission electron microscopy (4D-TEM), an advanced imaging technology that captures both spatial and temporal data at atomic resolution. This technique provided the evidence needed to validate their findings. By overcoming these challenges, the team not only advanced the understanding of twisted bilayers but also opened doors to more efficient, cost-effective ways of studying and utilizing 2D materials—relevant for future developments in electronics, quantum computing, and beyond.
The Versatility of Twisted Quasicrystals
The discovery of a vortex electric field in twisted bilayers comes with an unexpected bonus: the creation of a two-dimensional quasicrystal. Quasicrystals are structures that defy traditional classifications, exhibiting an irregular yet ordered arrangement of atoms. Unlike conventional crystals, their patterns do not repeat regularly, a property that gives them unique physical traits. For example, their low heat and electrical conductivity make them ideal for high-strength applications like surface coatings.
In this study, the quasicrystal emerged as a result of specific twist angles in bilayer molybdenum disulfide (MoS₂), which dictated the properties of the vortex electric field. The ability to control these angles and, by extension, the characteristics of the resulting quasicrystals has implications for technological innovation.
According to the researchers, these quasicrystals hold promise for enhancing memory stability in electronic devices, increasing computing speed through ultrafast data mobility, and enabling novel optical designs with polarizable effects. In spintronics—a field focused on using the intrinsic spin of electrons for information processing—the structures could facilitate dissipationless polarization switching, improving the efficiency of magnetic devices.
Beyond their immediate applications, this discovery also advances the development of quantum computing and spintronics. By resolving the challenge of cleanly stacking and twisting bilayers, the researchers have created a pathway for the next generation of quantum devices.
The study’s patented ice-assisted transfer technique further amplifies its significance, offering a reliable and cost-effective tool for exploring twisted bilayers in various materials. Future research will build on this foundation by testing whether stacking additional layers can enhance the vortex electric field or if similar effects can be achieved in other two-dimensional materials.
Small Twists, Big Impacts
Creating and manipulating vortex electric fields exemplifies how small iterations along continuous lines of development can have wide-reaching effects. From enhancing device performance to reshaping quantum technology, CityUHK’s research exists at the intersection of fundamental science and practical applications. As the team continues to explore the potential of twisted bilayer structures, their work may also support developments in electronics, quantum computing, and nanotechnology.
Contributing authors on the study include Chi Shing Tsang, Xiaodong Zheng, Tong Yang, Zhangyuan Yan, Wei Han, Lok Wing Wong, Haijun Liu, Shan Gao, Ka Ho Leung, Chun-Sing Lee, Shu Ping Lau , Ming Yang, Jiong Zhao, and Thuc Hue Ly.