Researchers Focus in on ‘Lightcone Bound’ To Develop an Efficiency Benchmark For Quantum Computers
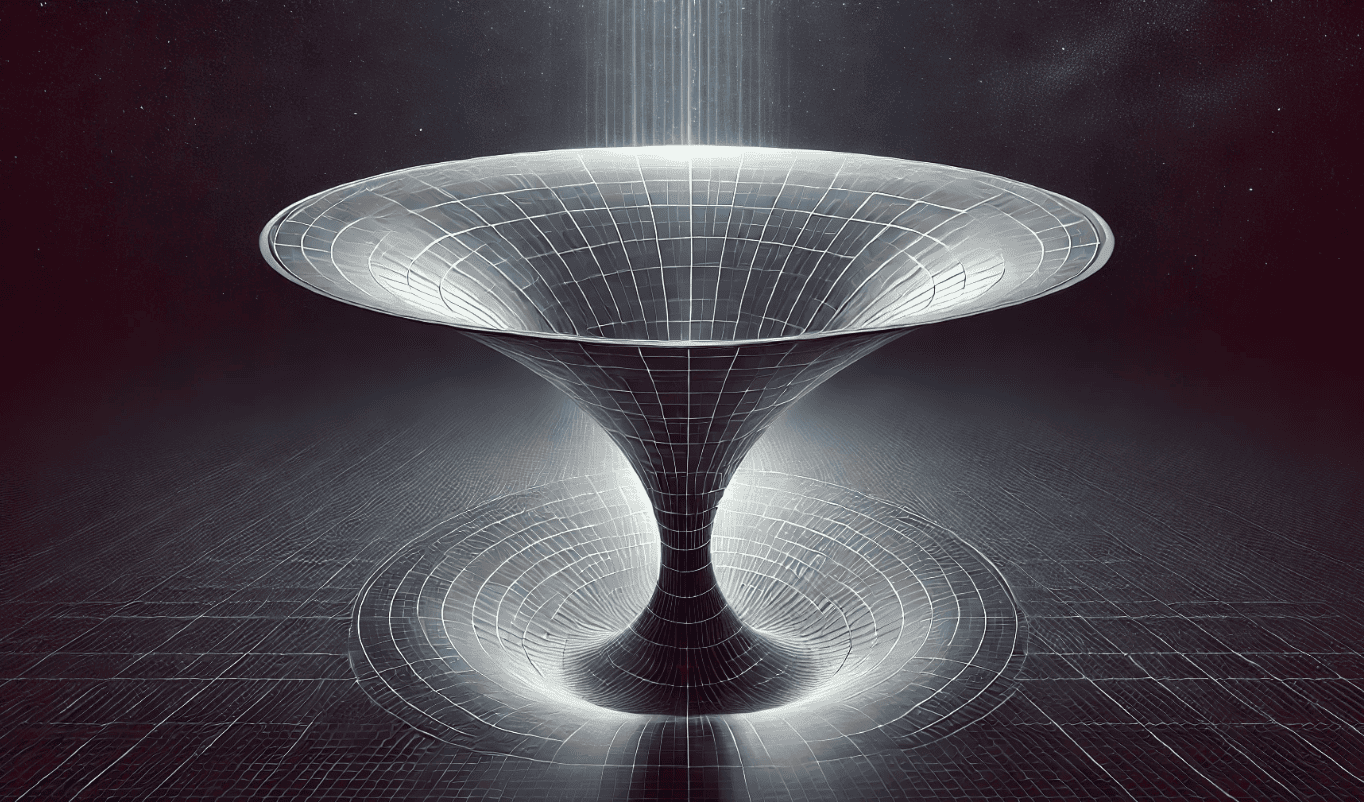
Insider Brief
- Researchers have introduced a “lightcone bound” that sets a new minimum overhead for executing quantum algorithms, offering a foundational efficiency benchmark for quantum computing.
- This bound minimizes the need for SWAP gates in quantum circuit mapping, which could significantly streamline the process of adapting circuits to specific quantum hardware.
- By reducing computational waste, the lightcone bound paves the way for more efficient quantum computing, potentially benefiting areas like cryptography and optimization in near-term quantum devices.
Quantum researchers have flipped the switch on a “lightcone bound” that sets a new minimum limit on the computational overhead for executing quantum algorithms on hardware — and that, the team points out, could potentially shape how quantum circuits are optimized, among other implications.
Writing in npj Quantum Information, the team explains that this work could define the lowest necessary overhead from SWAP gates — commonly used to adjust qubit placement — ultimately minimizing extra operations needed for mapping algorithms onto processors. The lightcone bound provides a baseline for circuit mapping on near-term devices and lays the groundwork for more efficient quantum computing methods.
The study, led by researchers from Delft University of Technology and the Technical University of Valencia, examines the longstanding quantum circuit mapping problem (QCMP), where circuit structures are adapted to fit specific processor architectures. Circuit mapping typically requires numerous SWAP gates, a time-consuming and resource-intensive process that can reduce computational accuracy.
This study takes a novel approach by employing principles from quantum information theory to set a lower limit on these extra steps, suggesting this bound could guide future circuit designs that aim to minimize that computational waste.
Lightcone Bound
According to the researchers, their lightcone bound is based on theoretical models from quantum information and graph theory. The researchers tested this bound by comparing it to IBM’s Qiskit compiler using 600 benchmarks, confirming the bound’s validity for reducing SWAP-gate count. By doing so, they established a reliable minimum requirement for SWAP operations, giving a benchmark for future quantum compilers.
To achieve this bound, the team developed its lightcone — a simplified model that assumes a noiseless, ideal quantum processor. This model operates under perfect parallelization, meaning that two-qubit operations — which is a standard quantum interaction — can happen simultaneously, reducing the need for rearranging qubits on the hardware. They also devised a novel initial qubit placement algorithm that uses graph similarity searches, matching the structure of a circuit to the hardware layout.
Apart from the primary bound, the study also reports on an initial placement algorithm that can arrange qubits in optimal positions before execution. By minimizing SWAP-gate requirements, this approach could make near-term quantum computers more efficient, addressing key challenges in circuit mapping.
This work also could inform the development of applications in quantum machine learning and other computational areas that depend on efficient circuit designs, the team reports.
Implications for Quantum Computing
As quantum processors evolve, the bound could become a standard for compilers, influencing the structure of future quantum algorithms. With minimal SWAP-gate interference, computations could be executed more accurately, potentially enhancing applications from cryptography to quantum optimization problems.
Researchers anticipate that this new bound will help circuit designers make better decisions when developing algorithms, particularly in applications requiring high precision and low error margins. Quantum machine learning, for instance, could benefit from the efficiency and reduced error rates enabled by such mapping strategies.
Methods
The researchers formulated this bound by first reinterpreting the quantum circuit mapping challenge through quantum information theory. They focused on the SWAP “uncomplexity,” the lowest number of SWAP operations needed, which they determined using graph theory and information geometry. By representing qubit interactions as density matrices, they applied concepts from network science to simplify circuit interactions.
To establish the bound, in an interesting twist, the team employed a Penrose diagram — a tool from theoretical physics typically used to depict spacetime geometries — to visualize the paths required for minimal SWAP-gate application. They then compared their model against a brute-force method and IBM’s Qiskit compiler, with consistent results affirming that their bound offers a practical minimum SWAP requirement for near-term quantum circuits.
Limitations And Future Directions
The researchers acknowledge the lightcone model has some limitations that could be the focus of future work. For example, it assumes ideal conditions, such as a noiseless processor and indefinite parallelization, conditions not yet achievable with current quantum technology. The model also does not account for single-qubit gate interactions, focusing only on two-qubit operations, which limits its direct applicability for certain quantum circuits.
As technology advances, however, the study’s findings could be refined to match real-world conditions.
The study suggests several avenues for further exploration. Researchers aim to improve the scalability of their qubit assignment algorithm, making it compatible with larger quantum processors. Another area for potential development is incorporating additional techniques, such as teleportation-based quantum circuit mapping, which could reduce SWAP overhead further. Future work could also focus on adapting the lightcone bound to error-corrected quantum circuits, which will become increasingly relevant as quantum processors scale up.
This study covers very technical information on quantum hardware and software that this article could not include, or could only summarize — for a deeper dive, please see the paper here.
Matthew Steinberg, Medina Bandić, Sacha Szkudlarek, Aritra Sarkar and Sebastian Feld, all of Delft University of Technology and Carmen G. Almudever, Technical University of Valencia.